Multiple regulatory changes combine to drive yeast’s ability to metabolize non-preferred sugars.
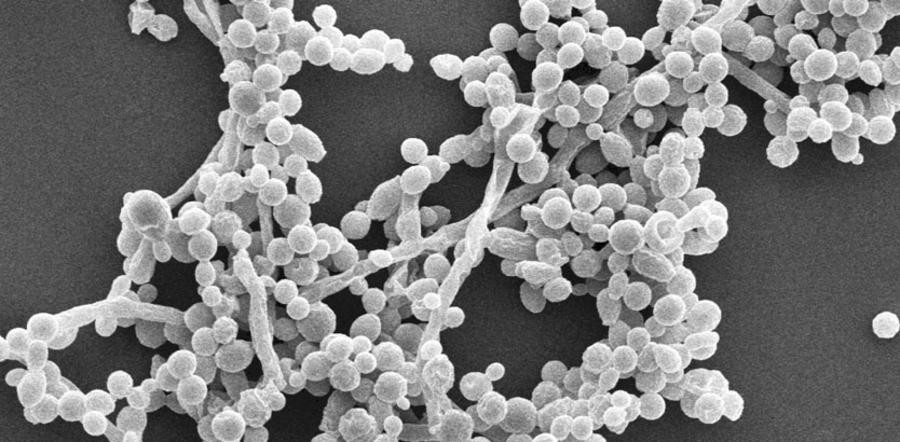
Five-carbon sugars such as xylose are abundant in plant biomass but are not readily broken down by the yeasts and other microbes used for biofuel production. Even yeast strains engineered to express xylose conversion enzymes fail to recognize xylose as a consumable sugar, suggesting that additional metabolic changes may be needed to drive efficient fermentation of non-preferred sugars.
Researchers at the Great Lakes Bioenergy Research Center (GLBRC) and colleagues at the University of Wisconsin–Madison investigated a series of yeast strains with varying abilities to ferment xylose anaerobically in order to explore the underlying physiological mechanisms that enable the evolved strains to convert xylose into biofuels. The work reveals that a host of regulatory changes and networks combine to mediate xylose fermentation.
The results suggest that widespread remodeling of cellular pathways, rather than individual metabolic changes, may be needed to achieve efficient biofuel production from xylose. Some of these changes may require rewiring regulatory networks in ways that decouple traditionally linked cellular functions such as growth and metabolism. A better understanding of cellular regulation of these functions may guide microbial engineering efforts to drive activity of desired production pathways while minimizing off-target effects.
Knowing that xylose conversion enzymes alone are insufficient to drive yeasts to break down the sugar at high rates, the researchers worked to dissect the broader metabolic and regulatory context needed for anaerobic xylose fermentation.
For this, they turned to a series of lab-evolved yeast strains that show progressive improvements in the ability to use xylose, either in the presence or absence of oxygen. The researchers applied multiple comparative systems biology approaches to identify transcript, protein, and phosphorylation differences across these strains that correlate with an improved capacity to use xylose. They also used a novel network analysis to infer the cellular regulatory networks most likely to mediate the observed phosphorylation differences.
The team found that changing the expression of individual genes had relatively small – though sometimes significant – effects. Instead, a suite of regulatory changes appears to be required for anaerobic xylose fermentation, with multiple downstream physiological effects combining to drive the phenotype.
The findings support previous evidence of the importance of protein kinase A (PKA) signaling, but show that different routes of PKA activation can lead to distinct outcomes. For example, deletion of the RAS/PKA inhibitor IRA2 drives both growth and metabolism on xylose, while deletion of BCY1, a direct PKA inhibitor, decouples growth and metabolism to allow robust xylose fermentation without division. In addition, the results reveal a surprising connection between the cellular responses to low oxygen and to the type of sugar present.
Together, the results highlight the challenge of predicting specific genetic modifications to engineer a desired outcome, as well as the importance of understanding the broader regulatory context of microbial metabolism to optimize biofuel production.