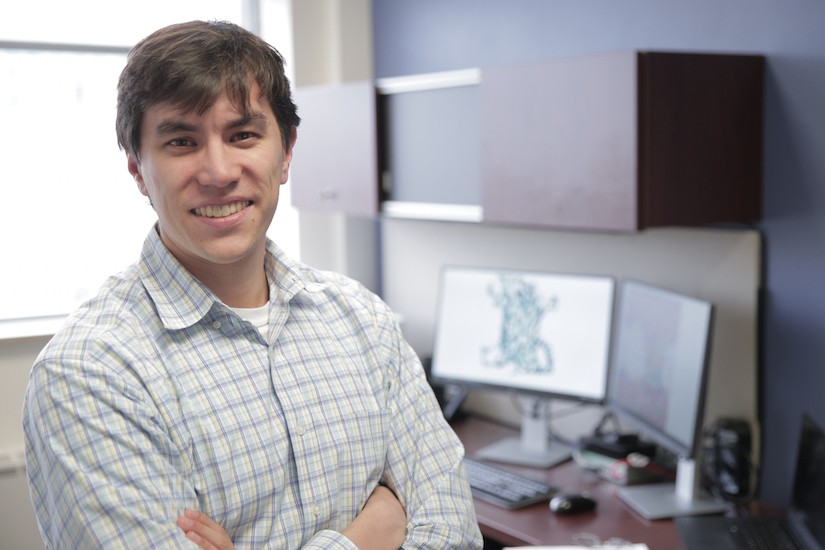
Advances in biofuels research tend to involve reduced costs, greater reagent stability, more diverse and valuable end products, or faster reactions, which often increase product yields as well.
But to understand at a deeper level exactly why one reaction happens faster than another, looking beyond the horizon of the biofuels world may be helpful—which is why Reid Van Lehn’s expertise in protein simulations led to an innovative research project with his colleagues and renowned biofuels experts George Huber and James Dumesic.
The researchers focused on a class of reactions that break down biomass by dissolving it in a mixture of water, a cosolvent and an acid catalyst. By modeling how tens of thousands of atoms move and interact with each other, they showed that the cosolvent speeds up the breakdown by concentrating water molecules in the physical space around the biomass molecules where the catalyst is active.
“Adding a cosolvent, which doesn’t even need to mix particularly well with water, dramatically increases reaction rates without having to change other conditions, such as temperature or pressure,” says Van Lehn, an assistant professor of chemical and biological engineering at the University of Wisconsin-Madison. “But what’s most exciting to me is that we can accurately predict these increases with computational models of atomic-level interactions between the reagents.”
With these models, published online on Feb. 8, 2018, in the journal Energy & Environmental Science, researchers can directly estimate reaction rates of newly designed biomass conversion processes from the solvent composition, without having to perform a series of expensive and time-consuming experiments.
The study is innovative for two reasons. It applied molecular dynamics simulations, which are often used for studying how proteins fold into 3D structures, to biomass-derived molecules, an entirely different class of chemicals. It also combined three particular quantities derived from these simulations in a statistical model that predicted, with high accuracy, the experimentally observed reaction rates.
Therefore, an entire class of reactions can now be analyzed in a much more high-throughput way, compared to the detailed theoretical calculations that had been used for one specific reaction in the past. “This demonstrates that we can treat a catalysis problem as a solution thermodynamics problem,” explains Van Lehn.
For the biofuels community, the new computational model may eventually become a commercial tool for process design, but more work is needed to get to that point.
“I think our study paves the way for optimizing biofuel production processes in the future by providing fundamental insights into the reaction pathways and developing methods for predicting their properties,” Van Lehn says.
Going forward, he also hopes to broaden the scope of the methods applied in this study to predict entire biological pathways, which could improve the design of drug delivery vehicles in medical applications.
The paper’s lead authors are PhD students Theodore (Ted) Walker, who is advised by Huber and Dumesic, and Alex Chew, who is advised by Van Lehn.