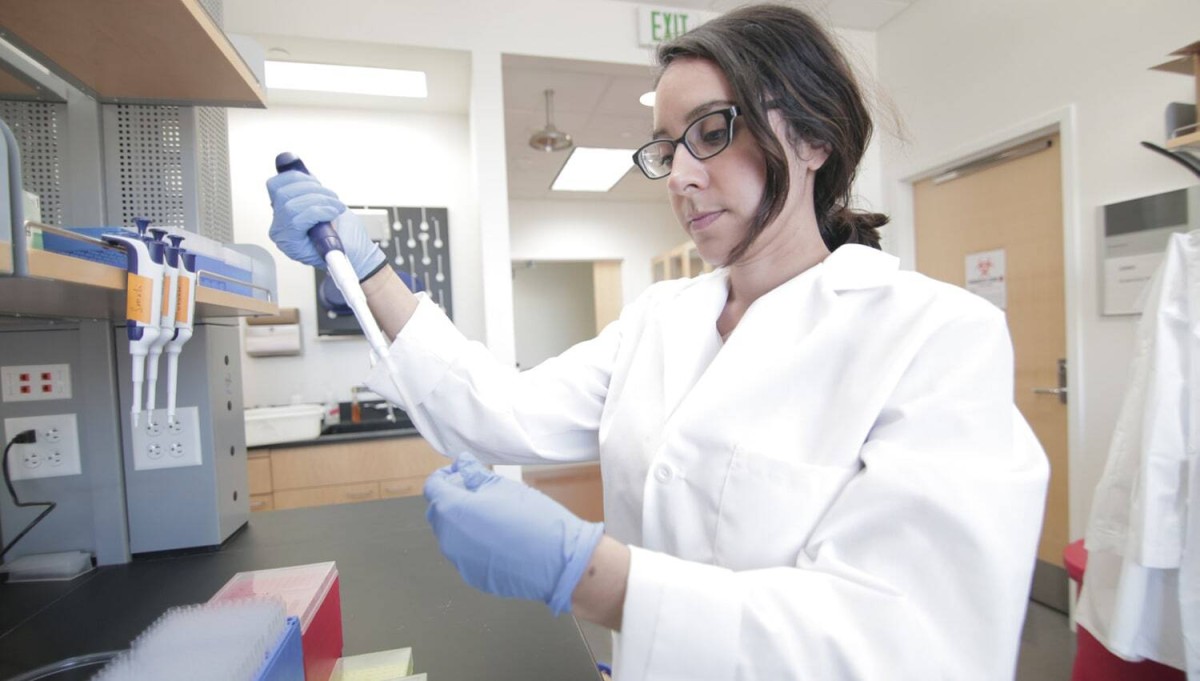
Microbial communities are everywhere in the environment. The interactions among these complex networks of organisms shape the overall community function and metabolism, sometimes in unexpected ways.
In a paper published May 31 in Nature Communications, a team led by University of Wisconsin–Madison assistant professor of biochemistry Ophelia Venturelli describes a generalizable, model-driven framework to predict microbiome growth and metabolic capabilities. Here, Venturelli talks about how they developed new computational models with an eye toward designing communities optimized for production of specific molecules, such as those useful for biofuel production.
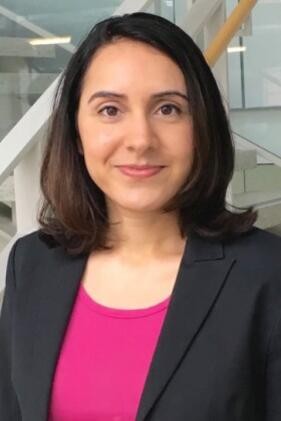
What is the power of microbial communities and why are they so useful?
Microbes don't ever exist in isolation. They compete for resources, produce resources that other microbes can use, and thus create new ecological niches or environments that sustain the growth of other organisms. And their collective metabolism is distributed—one microbe doesn't do everything, it's the integrated metabolic activities distributed across different constituent community members that determines the community impact on the environment. That environment could be a plant root, a human gut, the soil, or a bioreactor.
Our lab thinks about how we can design communities with tailored functions of interest. There’s an incredible diversity of chemical transformations that individual microbes can perform, and collectively they can achieve functions that are much bigger than the sum of the parts. We're trying to harness that capability for different applications.
What are the advantages of designing synthetic communities?
Natural communities are incredibly diverse, and there's a lot of really interesting work isolating natural communities from the environment and characterizing them in the laboratory. One challenge using natural communities is understanding mechanistically how a community functions—to identify the molecular, ecological and genetic control knobs for these systems, or to optimize a given chemical transformation or build a novel function that the community did not previously perform. For natural communities, there's a limited set of environmental parameters that you can control, like pH, temperature, and resources, which may limit our ability to precisely engineer properties of the community.
With synthetic communities, we have an additional control knob, which is choosing the organisms and changing the complexity or number of species in a community. With that control knob, we can start to understand the contributions of individual community members on a given physical or chemical transformation function. Then we can start to use computational models and high throughput approaches to vary the community composition and try to optimize or build a particular function.
How do you design a community to have the properties that you want?
This is one of the biggest challenges. There are so many different possible microbes that we could select to build a community.
We use an engineering-inspired strategy called a design-test-learn cycle, where you design a preliminary experiment to measure some aspect of your system, then use the resulting data to build a computational model that can suggest the most informative experiments to do. We go and do those experiments, then use that information to update our model.
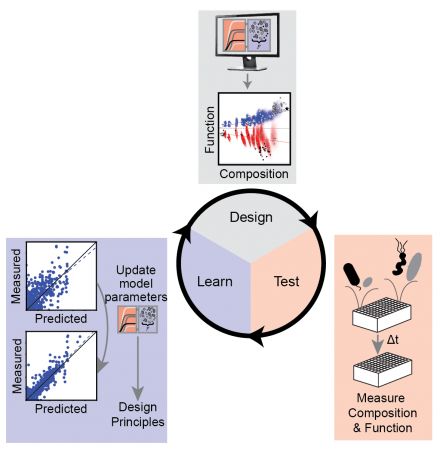
With the human gut microbiome in this paper, we started with a reductionist strategy where we looked at pairwise interactions between bacteria that we think are important in the system. We used those measurements to take a step forward and look at communities with three to five species—slightly more complex. And then we used all the information we had collected to predict the most complex communities that we could design, which had at least 11 species. With information about these more complex communities, our model was able to identify very high butyrate-producing communities and also identify the most important ecological and molecular mechanisms that were shaping the community functions.
It can lead to some surprises. You might think, put together the five specialized organisms that produce butyrate, and that community should be the highest butyrate-producing community. But by changing the other species present, we found communities that produced much, much more butyrate. Experimentally, it would have been really hard to find these communities—I think there's over 1 million possible communities that include all five butyrate producers—but our model allowed us to identify those specific communities with high butyrate production.
How does this work connect to the Great Lakes Bioenergy Research Center (GLBRC)?
This paper provides a foundation for how we can quantitatively link microbial community assembly to the production of an important metabolite, and then identify communities with high chemical transformation potential. Here, we focused on production of butyrate, but this approach is generalizable to other community functions. For GLBRC, we're working on developing model-guided approaches that allow us to identify communities that produce high levels of medium-chain fatty acids from conversion residue.
Ultimately, we want to be able to design new metabolic capabilities by leveraging synthetic biology in addition to synthetic community design and installing new functions in key species in the community. With both of those control knobs, then we can begin to design stable communities to perform important functions for diverse applications in human health, agriculture, and the environment.
Read the paper:
Clark R. L., et al. Design of synthetic human gut microbiome assembly and butyrate production. Nature Communications. 12: 3254. Published online May 31, 2021. https://doi.org/10.1038/s41467-021-22938-y.