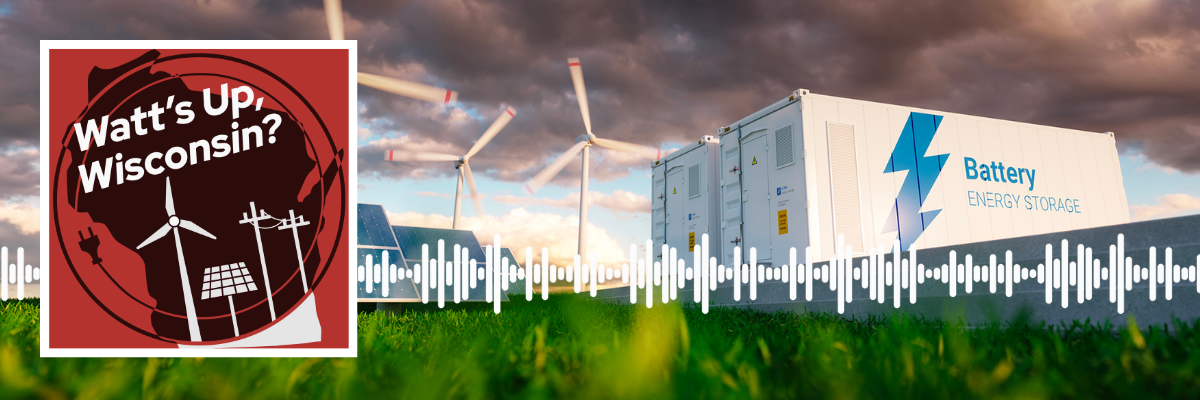
Solar energy is made during the day, but energy demand is highest when the sun sets. In order for intermittent energy sources, like wind and solar, to help in these peak periods, we need a way to store energy.
In this episode, we look at energy storage, understanding how we will store energy for a renewable energy future and what ducks have to do with it. We speak to battery researcher Eric Kazyak about how battery storage fits into this picture, from grid scale storage to electric vehicles.
Learn more: https://engineering.wisc.edu/clean-energy/
Do you have a question about energy in Wisconsin? Ask the Wisconsin Energy Institute at communications@energy.wisc.edu or tweet us and tag #WattsUpWI.
Listen to Watt's Up, Wisconsin? on Apple podcasts, Spotify podcasts, Google Podcasts, Youtube, or anywhere you find podcasts.
Guests
Eric Kazyak, Assistant Professor in the Department of Mechanical Engineering, UW-Madison College of Engineering
Eric's research is focused on making the way we convert, store, use energy more sustainable. His work is primarily experimental in nature, bringing together aspects of materials science, manufacturing, electrochemistry, and mechanics.
Credits
Britta Wellenstein, Host
Britta is a Wisconsin Energy Institute communications intern and UW–Madison undergraduate majoring in Life Sciences Communication and Environmental Studies.
This episode was produced by Britta Wellenstein and Michelle Chung.
Theme music by Eden Comer.
Transcript
Britta Wellenstein: When we think about using renewable energy as a large part of our energy grids, one issue can come to mind.
[duck quack]
Britta Wellenstein: That's right. Ducks.
[duck quack]
Britta Wellenstein: But not those kind of ducks. Well, exactly. The duck curve is a big issue in renewable energy. This graph shows the difference between electricity demand and solar energy production throughout the day. The graph starts in the middle when energy needs are somewhat high in the morning and those needs will drop midday and then shoot up at night.
But solar energy, on the other hand, is high during the day and not at night. This graph, if you squint a little bit, it kind of looks like a duck. But it represents a big issue with renewable energy. How can we supply energy during these peak demand periods when the sun isn't shining? One way to do that is by storing the extra renewable energy created in the day to use during the peak periods.
[music]
Britta Wellenstein: Today on Watt’s Up, Wisconsin, we're talking about how we store energy for a renewable power future and new technologies in battery storage.
[light switches on]
Britta Wellenstein: You're listening to Watt's Up Wisconsin, brought to you by the Wisconsin Energy Institute, where we explore your questions on energy in Wisconsin.
[cow moo]
Britta Wellenstein: I'm communications intern Britta Wellenstein. In order to keep up with renewable energy and maintain grid stability, we're going to need energy storage. The International Energy Agency, the IEA, estimates that to achieve net zero energy by 2050, battery storage will need to increase 35 fold by 2030.
Although batteries will be necessary, there are many nuances from the various types of battery is the economic and material limitations and social factors to consider. To learn more about this, I spoke to Eric Kazyak, assistant professor of mechanical engineering at University of Wisconsin-Madison. His research lab focuses on making energy storage more sustainable and alternative battery storage.
Britta Wellenstein: Then I'm going to ask very basic questions, and I think I can answer it. What is energy storage? Let's get a simple definition of what I would be.
Eric Kazyak: Sure. So energy storage at its core, or at least the way that we use energy storage for most purposes, is simply a way to decouple where energy is produced and where it's used. I would also add a caveat to that. Sometimes it's also when it's produced and when it's used. So shifting from when and where it's produced to when and where it's used. In some cases it's only when, and in some cases it's both when and where.
Britta Wellenstein: So it's kind of this middleman between where it's produce or when it's produced to when it's actually used.
Eric Kazyak: Absolutely, yes. So you're in some way capturing that energy and then you can use it at a later time or place.
Britta Wellenstein: What types of different energy storage are there?
Eric Kazyak: For this question, you could think about categorizing energy storage in a few different ways. One would be the mechanism by which that energy is stored. For instance, is that energy being stored in chemical bonds? Is it being stored in some mechanical way, for instance, just lifting up a heavy weight or pumping water up a hill? So that's one way the mechanism by which the energy is stored.
You could also think about categorizing energy storage by what it's being used for. Right. Are you storing energy to be used in a mobile application like an electric vehicle? Or are you storing electricity from solar when the sun shining and then discharging, say, a battery in the evening when the sun's not out or the wind's not blowing? So that's grid scale storage.
And then there's other types of storage, for instance, thermal storage that could be used to smooth out production. So some say a concentrating solar power plant heats up some fluid. Sometimes they use a molten salt during the day, and then that molten salt is still hot once the sun goes down. So they can continue using that to produce electricity so it can kind of smooth things out.
So that's another way we can categorize. You can do these all at once, really. There are not discrete ways of categorizing things, but you can also say, well, how long do I need to store that energy for? Am I storing energy on the timescale of milliseconds or seconds, like, say, a capacitor? Am I storing energy on the scale of minutes to a couple of hours, or am I storing energy on the scale of, say, more than 10 hours, 10 to 160 hours or so?
And those are all important. We need energy storage and all of those different categories in terms of timescales. But the technologies that accomplish those are going to be different or should be different.
Britta Wellenstein: You were talking about smoothing out the energy storage in reference to renewables. So what does that mean and how does energy storage fit in our renewable energy future with like wind and solar and other types?
Eric Kazyak: I kind of break up the role of energy storage in a, quote, renewable energy future into two categories. One is electrifying things that weren't previously electrified. So electric vehicles is a good example of that. But the other aspect is enabling us to use more and more of these intermittent renewable energy sources. So wind and solar, they don't produce the same amount of electricity all the time, right?
The sun's not always shining, the wind's not always blowing, and it's not always shining and blowing the same at every place in the country or across the world. Right? So that's very different from how we've historically generated electricity. In the past, we've had these kind of base production modes, largely based on fossil fuels. Nuclear and hydroelectric dams have also played a big role, but all of those are pretty much constant supplies of electricity.
We can ramp them up and down on demand, right? You can put more coal into a coal-fired power plant and ramp up the production to meet the demand from society, basically. But solar and wind don't do that. So you need some way to be able to shift the production of electricity in time, right from when you have sun, when you have wind to when you need to use that electricity.
So that's a key role. And in the past we have had some energy storage on our grid and it's largely been in the form of pumped storage, hydroelectric, and it's represented roughly 2% of our total generating capacity has been in the form of this pumped storage hydro electric, where you have a hill, you have a body of water at the bottom and the top of the hill.
When you have excess electricity, you pump the water up the hill and when you need a little bit of a boost, you let the water flow down the hill through a turbine and generate electricity. And that has helped with things like peak shaving. So the last little bit of electricity production, the maximum amount of power is super expensive, right?
Because if you're using a traditional power plant to generate that last little bit of electricity, then you have a power plant that's only operating a very small fraction of the year. So that's very expensive to do. So then by implementing just a little bit of storage, you could help smooth that out. Even with these traditional types of generation, but with renewables, we're going to need a massive amount of storage compared to that much larger amounts of storage.
And we can't just build more pumped storage hydro electric. In the last 20 years, we've done almost none of that because it's very geographically constrained, right? There's not a lot of places that you have a big hill to bodies of water near a power plant, basically. So that's where various different types of energy storage. So I'm a battery guy. If you wanted to describe me in two words, but I appreciate that batteries are only a part of a portfolio of different types of energy storage that will play a role.
Britta Wellenstein: This peak demand period Eric was discussing makes up part of the duck curve, which storage can help us overcome. Although pumped hydro is the most popular type of storage, battery storage is increasing. Grid scale battery installations rose 75% from 2021 to 2022, according to the IEA. But this rise has relied on one type of battery storage, and it's probably in the device you're using to listen to this right now: lithium ion batteries. Lithium ion batteries have boomed in the past decades. They are what power the majority of our lives from our phones, our laptops and even some electric vehicles. But as Eric sees it, they're not a solution for everything.
Eric Kazyak: The way that I see it, we're kind of shoehorning certain technologies into all of those different applications because it's what we have rather than it being the best option.
Britta Wellenstein: Could you give an example of that shoehorning?
Eric Kazyak: Yeah. So so lithium ion batteries have made huge strides in the last ten years or so from 2010 to 2020, we saw a 90% reduction in cost of lithium ion batteries. And because of that, they've become economically viable for a wide range of applications. Electric vehicles is probably the best example of that and it was the driver for that.
So companies like Tesla scaling up the production of batteries to a massive scale and streamlining the supply chain to a certain degree really enabled bringing down the cost of lithium ion batteries. But because of that, because lithium ion batteries were kind of out front in this next generation of energy storage, they have a cost advantage compared to some of these other chemistries, and that enabled them to get a foot in the door for things like grid scale storage.
So I mentioned smoothing out the production from things like solar and wind and lithium ion batteries. They're very good at storing a lot of energy in a small amount of space, but they're not necessarily the best fit for grid storage in my view, because for grid storage, you don't necessarily care about how much the thing weighs or how big it is.
If it's just sitting in a container somewhere next to a wind turbine or a solar farm. Right? So those aren't really strengths in that application. But because it was first, lithium ion batteries are the easiest to get banks to finance for grid storage applications. Currently, and it's starting to change a little bit. We're starting to see other types of batteries being implemented for demonstration scale projects, and by demonstration scale, I mean very large demonstration, so up to gigawatt hours scale batteries that are not lithium ion batteries.
Britta Wellenstein: As Eric pointed out, lithium ion batteries have become incredibly common, both for grid storage and for cars. Lithium ion batteries work by moving charged lithium ions through a liquid from one side of a battery to another. This movement produces free electrons, which creates an electrical current that powers our devices. What makes us so great is that this mechanism allows us to recharge our batteries.
This works great for smaller devices like our phones or laptops, but is more difficult for larger scale devices like cars or grid storage.
Britta Wellenstein: I'm going to switch a little bit to more of your research specifics, which is in two batteries and lithium ion batteries and other alternatives. So lithium ion are in like everything. They're in probably the mic we're using right now, the computers we have, our phones, they're the basis of kind of our electronic world. But your research has highlighted a lot of issues with lithium ion. So can you go over some of those challenges?
Eric Kazyak: One of the things that I love about working in this field is that most people, as you said, are interacting with devices that use batteries on a daily basis. So we kind of have an intuitive grasp of a lot of the challenges that I'm trying to overcome in my research. One of those would be fast charging. So how long does it take a device, whether that's your cell phone, your laptop or an electric vehicle to go from almost dead to almost fully charged?
If that were 10 seconds? Right, we wouldn't even think about it. But it's not. We know that it takes longer than that to charge these devices. And for a cell phone, it's kind of a minor inconvenience in most cases, right. On a regular basis, at least me and most of the people I know, you kind of charge your phone overnight and then it works for most of the day or all day.
Right. But an electric vehicle, that's the use case for a lot of the time. If you're commuting to work or just going out to get groceries or whatever, those short trips, you can kind of use the electric vehicle that way where you don't necessarily have to think about charging if you're in a situation where you can charge at home.
But that's not everybody. People that live in an apartment or various other reasons they might not be able to charge at home. So in those cases, you're going to want to be able to go to a charging station, a publicly available charging station, and plug in your car and not have to wait 8 hours. Right? That's yeah, that's not tenable in my mind.
And that could be true for kind of your day to day life, right as you're going about your day, but also where it probably becomes the most of concern for the majority of people is on a road trip. If I want to drive somewhere that's further away than the range of my electric vehicle, then you really start to think about, well, how long am I going to have to stop and charge this thing?
Now, the first generation of electric vehicles were very slow at charging, and that was partially due to the batteries. It was also partially due to the infrastructure side. There just weren't charging stations that could supply enough power. We're talking about serious amounts of power here, tens to hundreds of kilowatts. So in many cases, larger than the entire electrical connection to most houses is the amount of electricity that a fast charger uses.
So fast charging is key. I hear some talk in my field like we need to make batteries and battery electric vehicles, more specifically, charge as fast as you can refill internal combustion engine vehicle with gasoline, right. So that's 2 to 3 minutes, maybe up to five if you're generous. The problem with that, in my mind is, like I said, this is part of a system, right? These devices don't exist in a vacuum. And when you're talking about an electric vehicle, you're talking about 50 to 100 kilowatt hours of battery, at least. Now, some of them are you know, the Hummer EV is 200 kilowatt hours of battery.
So, for instance, if you wanted to charge a 100 kilowatt hour battery, that's roughly a 300 mile range EV If you wanted to do that in 3 minutes to match internal combustion engine, you would need two megawatts of power. Again, for context, a typical house only has a 20 to 40 kilowatt connection. So there would be a very select few places right now, at least on our infrastructure, that could even support that level of charging. And beyond that, the cable that you would need to pass that much current would weigh so much that humans wouldn't be able to pick it up and put it in the car, so you would need a robot to do that. So it becomes untenable very quickly in my mind once you get past, say, 10 minutes of fast charging. So that's where I think we need to be. Somewhere between 10 minutes and 15 minutes to go from, say, 0% or 5% of battery to 80%. The last 20% of charging gets really hard to do it quickly for kind of deep physical reasons intrinsic to lithium ion batteries.
Now, some of the next generation chemistries that are coming online soon, solid state batteries, for instance, can overcome some of the intrinsic challenges with fast charging. So you could possibly enable faster charging rates than that, particularly, maybe pulse charging and say, I can add 100 miles in 3 minutes or something like that. You might be able to do with solid state batteries, so there are some exciting things on the horizon.
Britta Wellenstein: There are various alternatives to lithium ion, both for grid storage and for electric vehicles. One option is solid state batteries. As Eric mentioned, solid state batteries work in a similar way to lithium ion. Instead of the lithium ions passing through a liquid, in solid state batteries, they pass through well, a solid, which is denser and can hold more energy for longer. Flow batteries are also becoming increasingly popular for grid scale storage flow batteries use electrochemistry and the transfer of electrons between cells to store electricity. These tanks or cells can be scaled up or down to store more energy and stored for longer.
Eric Kazyak: For transportation, the big up-and-comers in the industry are so sodium ion would be the low cost and lithium ion phosphate is kind of an intermediate. Then there's our current state-of-the-art lithium ion. We also have alternatives that are 3 to 5 years out for the high end of performance and currently they're too expensive for full EVs.
So Toyota relatively recently announced that they will be putting a solid state lithium metal battery. So not a lithium ion battery.
So the reason why lithium ion batteries are called lithium ion batteries is this this harkens back 40 to 50 years when the lithium ion battery was discovered. So there were people working on trying to store energy. Right, and they were trying to use lithium metal to do that. Lithium metal is very reactive. So it causes a lot of problems when you try to make a cell out of it. They just don't last very long. It tends to form what are called dendrites, these kind of tree looking structures that short circuit the cell and the major, you know, eureka moment was when they figured out that they could store lithium in its ionic state rather than going all the way to metal, so you can essentially shove these lithium ions between the layers of different materials, graphite being the one that was closest lithium metal. So it works really well. That's still what we have largely in our devices today is graphite, and it works well because, you know, the reason why we use it in our pencils is because it's this layered material and these layers can kind of sloughed off and the trace that's left behind from the pencil, that's those layers of graphite that are being broken off.
So in the lithium ion battery, you can shove the lithium in between the layers and then you can pull it back out very reversibly you can do that thousands of times in some cases without losing much capacity. But now, you know, we're kind of sort of approaching the limits of what lithium ion batteries can do, theoretically.
We're always developing new materials, so we're not at the limit, I'm not saying that. But the key enabler here is we can replace the flammable liquid electrolyte that's in today's batteries with a solid state electrolyte, that's non-flammable. It's a ceramic actually, it's high temperature stable. And in doing that, you can use the lithium metal electrode, which has on the electrode level about ten times the capacity per weight as graphite, which at the cell level or the pack level translates to 2 to 3 times as much energy.
So now we're bringing into play things like having an electric vehicle that has a 700 mile range or 800 mile range and it overcomes some of the intrinsic challenges to fast-charging lithium ion batteries because instead of trying to shove these lithium ion batteries between layers of graphite, which you might imagine, they tend to pile up at the surface and then bad things happen.
In a solid-state lithium metal battery, you actually are plating lithium metal intentionally. So nothing different is going to happen. There are bad things that can happen. It's less bad that they don't catch on fire. I won't go into too much detail there, but there are alternatives. So I mentioned Toyota–they're going to put these solid state batteries into a hybrid vehicle in the 2025 timescale and several other automotive companies are planning 2028 timeframe for these solid state batteries going in full electric vehicles so they're scaling up, building large factories. So there's a lot of industry interest in this space right now. That's really exciting.
Britta Wellenstein: However, lithium ion and these other battery chemistries use critical minerals. These materials like copper, cobalt, nickel and lithium, among others, are relatively rare and have a range of problems associated with their mining, including environmental damage, loss of biodiversity, greenhouse gas emissions and social impacts like the mistreatment of workers and child labor. But critical minerals are necessary to keep up with renewable energy goals.
Electric cars alone have increased in sales by 40% in 2020. EV and battery storage are expected to account for half of the mineral demand growth in the next two decades, according to the IEA. But researchers like Erik are working to find ways to limit the amount of critical minerals needed and are working to limit these social impacts.
Eric Kazyak: There's a number of different materials that go into lithium ion batteries that are problematic when it comes to environmental impact, social justice impacts, and cost as well. So the three big ones right now that are all defined as critical materials by the federal government, lithium is the obvious one. Cobalt is the most problematic one on a per gram scale, and then nickel is increasingly becoming problematic.
So about ten years ago, almost all the batteries with lithium ion batteries that were being produced were lithium cobalt oxide base. The cathode material was lithium, cobalt oxide. And we quickly realized that cobalt, which was coming from the Congo, which is extremely problematic from a geopolitical standpoint, from a social justice standpoint, from an environmental impact standpoint, from a cost standpoint, really bad. So quickly, the industry started pivoting and figuring out ways that we could reduce the amount of cobalt going into these batteries.
We did that largely by increasing the amount of nickel in these batteries. We were replacing cobalt with nickel. As a result, we did successfully reduce and in some cases now we're even fully removing cobalt from the batteries. But nickel is now becoming a huge problem, both from cost and impact standpoints for the nickel and cobalt side, there are alternatives.
So Tesla's started putting lithium ion phosphate into their economy level vehicles, their model threes, because it completely removes the nickel and cobalt from the battery at the cost of slightly reduced performance, but in my mind it's definitely worth it. It still has a lithium in it, but some other problems. There are also alternatives for lithium. They're completely different chemistries.
But sodium ion batteries are now becoming a more common in the space, not the weight of no vehicles in the U.S. yet that I'm aware of, at least that are using sodium ion batteries. But in Asia, there are now vehicles coming online with sodium in batteries. The performance won't be as good. You won't have as long of range, but that will help overcome some of these challenges.
Britta Wellenstein: Another option for long term grid storage is ion air batteries, which is being researched at form energy in Minnesota. We all know ion from probably its most annoying property rust, but we can make energy from that chemical reaction.
Eric Kazyak: So it's essentially reversible rusting. They made an electrochemical cell where they can instead of just, you know, having a piece of iron rust out in the open air, they split apart the two half reactions that are involved in rusting. So then they can get voltage out of it, and then they can drive it the other direction, too.
If they apply a voltage, you can strip the oxygen off of the iron, produce oxygen and iron. So that's really exciting because iron is very earth abundant. Right? And oxygen obviously is everywhere. So in theory you could scale this up to a really, really massive scale. Now that, you know, the technology is not nearly as mature as lithium ion batteries.This is one of their early demonstration scales. But hopefully this will prove that this can be done at the scale, it can be done cost effectively, safely. That's another benefit of the chemistry. It's intrinsically safe, not flammable. It's what would extinguish a fire if it was in the building. There's a lot of exciting stuff going on there.
Britta Wellenstein: Despite the complications in the battery storage industry, Eric is optimistic for the future. He's alongside a new cohort of researchers at the College of Engineering at UW–Madison looking into energy storage. They work as a part of the college's Clean Energy Community Initiative.
Eric Kazyak: There's few of us that work on batteries. There's hydrogen fuel cells and electrolysis. There's various different areas within energy storage, and they're throwing a lot of resources and support behind that. So over the next couple of years, I'm really excited to see what comes of that. And we're now engaging with industrial partners. So different companies in the state of Wisconsin and around the country as well that are really interested in supporting energy storage research and kind of having an exchange of ideas here at UW–Madison.
The optimistic outlook here is that there's a lot of very smart people working on a lot of different, very promising technologies that all have a role to play in this future sustainable society. It's not going to be some silver bullet that comes in. It's not lithium ion batteries that are going to solve our all our problems. Lithium ion batteries have a role to play in the future, but so does thermal storage, so does flow batteries, so does hydrogen. And they all just need to be used in the way that makes the most sense. Right?
We need to be mindful and intelligent about when and where we use each of these technologies, but I think the outlook is very positive in terms of getting to where we want to go because of all of these promising technologies and not just the technologies, but also the people that will be using them in smart ways.
[music fades in]
Britta Wellenstein: Thanks for listening in. Do you have questions about energy? Wondering how new energy solutions are taking off in Wisconsin? Let us know by sending an email at communications@energy.wisc.edu or tweet @uwenergy and tag #WattsUpWI.